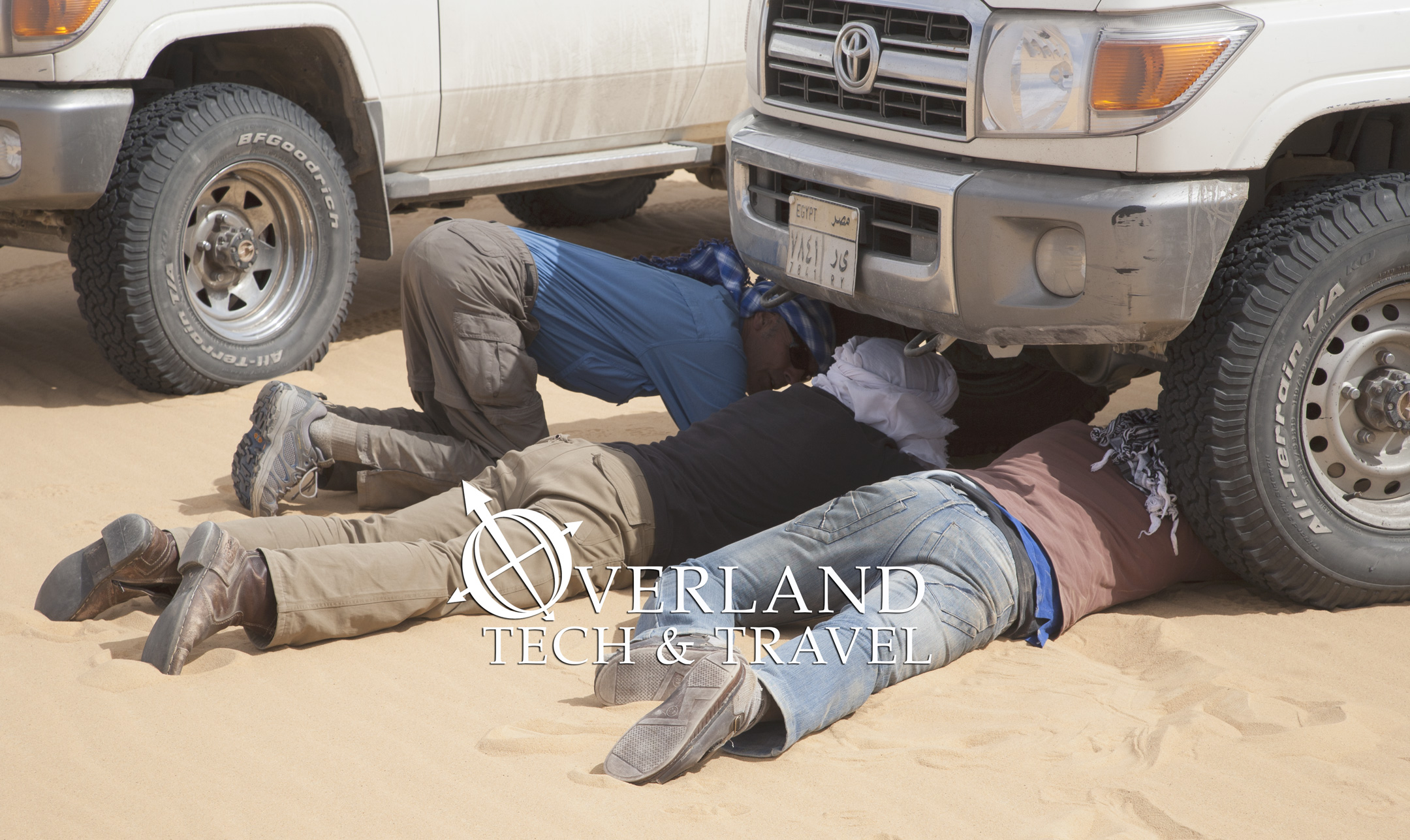
Overland Tech and Travel
Advice from the world's
most experienced overlanders
tests, reviews, opinion, and more
Hella horns
The only car I’ve ever owned that had decent factory horns was a Porsche 911SC, which was equipped with proper Autobahn blasters to move inattentive slower cars into the right lane right now. All others—and especially all the Japanese makes—needed serious upgrades to perform at a level I consider acceptable. I don’t use my horn very often, but when I do I want it to capture its target’s undivided attention. This has become significantly more important in the age of texting—which studies have show to be more dangerous than drunk driving. Why? Drunk drivers, at least, are generally paying attention because they know they’re drunk and they don’t want to alert the police. Texting by definition requires one to remove all one’s attention from the road.
Thus all of our vehicles carry Hella horns of one model or another. They’re affordable, starting around $20 or so, and all have an authoritarian tone. My favorites are the twin or triple-trumpet air-powered models, which have a lovely teeth-on-edge screech, but the lesser models such as the ones here are still excellent.
These went on Roseann’s mother’s RAV4, which we have taken over since she gave up driving at 90 and is now allowing us to chauffeur her around town. The stock horn on this vehicle was even worse than most Japanese horns, which tend to sound like sheep bleating. This one sounded like an asthmatic sheep bleating.
Of course, being a modern vehicle, removing the “grille” on the RAV to access the horns actually involved peeling off the entire plastic front end of the thing . . .
. . . which, thanks to a helpful YouTube video describing the location of the hidden fasteners, only took about ten minutes.
After that it was easy. The Hellas require a separate ground wire, which I simply connected to each horn’s mounting bolt. The result is a huge improvement. I’m going to go hunt for texters.
Buyer beware: Fake NATO fuel cans
A properly constructed NATO fuel can. Note the full welds on the handle, the fully recessed perimeter weld, and the reinforced spout.
I can smugly claim to have been a very early adopter of the NATO jerry can—at least in terms of U.S. overland travelers—after becoming disgusted with the poor design of the U.S. Blitz cans with which I originally equipped my FJ40’s rear rack. The Blitz cans leaked at the screw-in lid, they seeped at the crimped seams, and they took forever to decant into the main tank via the poorly ventilated flexible spout. I loathed the things but it was all we had. Or so I thought.
Some time in the mid-1980s, after noticing NATO-style cans in photos in various articles (including, IIRC, one by Tom Sheppard), I did some research, and began to realize how superior the original Wehrmacht design was. The Brits and, later, NATO were smart enough to copy exactly the design of the German cans they liberated during the North Africa campaign. The perimeter seam was welded, not crimped; the lid was a small-diameter hinged assembly that cammed tightly closed; a generous breather and properly designed spout made decanting a fast and leak-free procedure. The interior was lined with a rust-proof coating.
For misguided reasons of economy the American military, with their own Wehrmacht can to copy, decided to cut corners, and the result was the hugely inferior Blitz can. In fact the only effective feature we adopted was the three-handle design, made so one soldier could easily carry four empty cans, or two full ones, and so a line of men could easily pass full cans along without fumbling.
I ordered two surplus NATO cans from overseas at absurd cost—and never looked back. They didn’t leak, even when stored on their backs or sides, even in 110º Arizona sun. Decanting was easy and fast. The improvement over Blitz cans was night and day.
Wide breather orifice aids fast decanting.
Surplus NATO cans, and current production models from NATO suppliers, became easily available after the turn of the millenium. Wavian now imports new NATO cans directly from the Latvian factory where they have been made since WWII. (Valpro cans are identical.) Other high-quality NATO cans are available from Gelg (Polish manufacture), although they are made of slightly thinner steel—.8mm versus the .9mm of Wavian/Valpro.
My later testing against the newer U.S. military (plastic) Scepter cans only reinforced the NATO can’s supremacy. Every other Scepter can I tried seeped at the wide lid. You could buy any of a number of special spanners designed to crank the lid as tight as possible, and get it open again—a bandaid “solution” to a poor design. The plastic sides bulged in heat and jammed in their holders. Besides which, being plastic, all Scepter cans are doomed to become plastic trash at some point. Thus, the NATO jerry can remains my fuel can of choice.
However, recently I’ve been noticing Chinese copies of the NATO can that are shockingly inferior to the European-made versions. You can find them on Amazon under the brand name Teekland, for one, and at Walmart (and, I presume, other discount stores). At a glance they look to be identical to their more expensive counterparts, but even a cursory inspection shows this to be a sham. The steel is thinner than the Gelg cans—.7mm. The interior is uncoated and rusts instantly. The handle is attached with spot welds rather than strip welds. The breather is a tiny tube rather than the generous orifice of the Wavian/Valpro and Gelg cans. Also, critically, the welded perimeter seam is not recessed as it is on authentic NATO cans; thus you cannot effectively store these cans on their backs. Absolutely nothing about these ripoffs except the styling is the same as the European-made containers.
Chinese copy. Note the tack welds on the handle, the raised perimeter weld, the flimsy and poorly welded spout, and the undersized breather tube inside.
But there’s worse. The online Walmart ad claims a list prince of $169.99, selling for “only” $64.99. Yet even the premium Wavian can lists for $80; the Gelg for $50. Thus Walmart will sell you an inferior fuel can for more money. (The Amazon can is $50; identical in price to the distinctly superior Gelg.)
So, caveat emptor—look for either genuine NATO surplus jerry cans, or newly manufactured ones from either Wavian or Gelg.
Redarc and Antigravity
As I mentioned here, LiFePO4 batteries have a different charging profile than lead-acid batteries. For one thing, they can take a much faster charge. However, they also don’t like to be constantly trickle-charged. Thus for an installation that replaces an AGM auxiliary and starting battery system, as I’m doing with our Troop Carrier, I also needed to replace the existing charge controller.
The choice was pretty easy. The Australian company Redarc, while fairly new to the U.S., actually has a 40-year history in its home country, and thus extensive experience building vehicle electronic systems capable of surviving hostile Outback conditions. I know a half-dozen people personally who rely on Redarc charging systems in their vehicles, from a Hilux to a Mitsubishi camper, and none has had a failure.
After a consultation, the company sent me one of their BCDC1240D controllers, an RK1260 relay, and all the necessary connectors and fuses.
This system will be able to integrate the input from the Land Cruiser’s alternator along with that from its two 100-watt Renogy PV panels, to keep both of the Antigravity batteries properly maintained.
I’m very close to tackling the installation; just waiting on a couple of custom cables and an aluminum heat shield.
LiFePO4 vs. lead-acid starting batteries . . . on a bathroom scale
An impressive difference—a friend noted that the standard battery for a Honda Gold Wing motorcycle weighs 13 pounds.
LiFePO4 battery: cylindrical cells or prism cells?
If you research LiFePO4 starting and deep-cycle batteries beyond the glossy ads, you’ll soon uncover the two major types of component construction used in their manufacture: cylindrical cells or prism cells.
Cylindrical cells look just as their name implies. Cut open a battery built with them and it will look like several dozen standard D-cells, connected end to end and arranged in rows and columns.
Prism cells for automotive use are larger and look more like flat bricks; typically such cells are 3.2 volts each, thus four of them connected in series create a 12.8-volt battery. (This is how Antigravity Batteries builds their LiFePO4 units.)
Which is better? Not surprisingly, manufacturers using cylindrical cells say cylindrical cells are better, and those using prism cells say prism cells are better. In truth, a high-quality battery can be built using either construction. The difference is in details, and the emphasis each manufacturer places on the respective characteristics of each type.
Cylindrical cells are more amenable to volume production, and are thus less expensive. They handle internal pressures well, and the multiplicity of cells in a typical battery means that if one cell fails, the battery can continue to operate. However, they take up more space, and are more quickly affected by temperature swings—since each cell is small and has an air space around it, they will heat up more quickly or drop below a critical lower temperature more quickly. (On the other hand, of course, they will recover from over- or under-threshold extremes more quickly as well.) A battery made with cylindrical cells necessitates a multitude of mechanical connections to build the completed unit, increasing the potential for internal structural issues due to vibration and impact.
Prism cells are more difficult and thus expensive to manufacture. Their chief advantage lies in a greater ability to tolerate over-charging as well as over-discharging. Thanks to their configuration they also boast superior energy density compared to cylindrical cells, which means greater flexibility for the manufacturer, and potentially lighter weight in the completed battery. In terms of thermal management, their compact form means a prism-cell automotive battery will take significantly longer to reach either a high or low temperature threshold—and, of course, longer to recover from that threshold.
Several configurations of prismatic LiFePO4 cells
According to Scott Schafer at Antigravity, the company’s first LiFePO4 batteries were cylindrical-cell models—but they switched to prism cells some time ago. Why? “Reliability,” according to Scott. He noticed that the higher-end manufacturers of cells were using prism technology more and more, and indeed, since switching their offerings, Antigravity has experienced a drop in warranty claims, which he attributes largely to that greater tolerance for over-charging and over-discharging. That was enough to convince me.
Be your own optics expert
What could be more magic than an invention that brings the world seven, eight, even ten times closer? A shy bird, a dangerous animal, the moon and planets, a distant and questionable 4x4 route (or, in the above photo, a possible poacher): a thousand things can be enjoyed, evaluated—or apprehended—with binoculars.
I’ve used binoculars for fun for many decades, and I never tire of simply sitting and looking through them at anything that catches my fancy. However, I’ve also used them professionally, as a sea kayaking, natural-history, and birding guide, and the latter experiences in particular have taught me a lot about both choosing and using them the most efficient way. Like many products, to a large extent you get what you pay for—but there are ways to ensure you get the best instrument for your needs regardless of how much you plan to spend.
(I’ll note here our weird misuse of the term “binocular,” which means, of course, two oculars. So technically we look through a binocular, rather than a “pair of binoculars,” which would be something both you and a friend could look through at the same time. However, the term is so ingrained you’ll probably catch me using it in the next paragraphs.)
Binocular anatomy
The single most visible diagnostic component of a binocular is the front, objective lens. All other aspects of construction being equal, the diameter of this lens determines, 1) how much light the binocular gathers and how bright the image appears, 2) how much magnification the binocular can effectively deliver, 3) how bulky and heavy the binocular will be to carry and use, and 4) how much it will cost. Small—e.g., 20 or 25mm—objectives equal low light-gathering, low power, light weight, and lower cost. Larger—30 to 60mm—objectives gather more light, allow higher magnification, are heavier and bulkier, and cost more.
Objective lenses. Left to right: 20mm, 32mm, 42mm
Remember that as objective diameter increases linearly, objective area increases geometrically. The 32mm objectives in the middle in the photo above have two and a half times the light-gathering power of the 20mm objectives on the left.
Binoculars are referred to by their magnification factor (the number of times they make an object appear closer) followed by the objective diameter—6x20, 8x42, 10x42, 10x50, etc. The compromise between objective-lens size and magnification is one of the main decisions you’ll need to make when selecting a binocular. So let’s look at the relationship.
Hold a pair of binoculars a foot or so from your face. See the little circle of light in the eyepiece? That’s called the exit pupil.
The diameter of that exit pupil is a function of the objective lens diameter and the magnification of the instrument: objective diameter divided by power gives you the exit pupil diameter—in any binocular, regardless of construction or cost. A 10x40 binocular has a 4mm exit pupil; so does an 8x32 binocular. An 8x40 binocular has a 5mm exit pupil, in a compact 8x20 binocular it is 2.5mm.
Why is this important? It has to do with the variable diameter of your own pupils as they react to light. In bright sunlight your pupils will contract to just 2mm or so, but near dusk they will expand to 5, 6, or even 7mm, depending on your age (as we age our pupils cannot expand as much). Consider that compact 8x20 binocular and its 2.5mm exit pupil. Looking through it in bright sunlight, with your own pupils constricted to 2mm or so, you’ll see a full, bright view. But look through it near dusk, when your pupils have expanded to 5mm or more, and you’ll see dark clouding around the central image, because your pupils are larger than the exit pupil. This vignetting means that binoculars with small exit pupils don’t perform well in low light (which can also include a shaded forest, for example). Thus binoculars with larger exit pupils are better for viewing near dawn and dusk. Years ago I had a pair of 7x50 binoculars with a stupendous 7mm exit pupil. Despite otherwise modest optical quality they excelled at low-light viewing.
Comparison of the exit pupil of an 8x50 binocular with an 8x20.
So, all else being equal, a larger exit pupil equals better low-light viewing. However, magnification has an effect as well. Note the 10x40 and 8x32 binoculars I listed above. Despite identical 4mm exit pupils, the 10x40 will perform better at dusk due to the greater magnification. (As we’ll see, many other factors also contribute to brightness.)
Herewith my first guideline: For all-around use I do not recommend a binocular with an exit pupil smaller than 3mm, and 4mm is notably better. Those 10x25s in a company’s catalog might seem like a wonderful combination of high power and compact size, but their 2.5mm exit pupil will be a serious hindrance in low light.
The same binoculars, showing relative size. These are all roof-prism instruments—note the oculars in line with the objectives.
Now let’s look at the next most important feature of binocular construction: the prisms.
All modern binoculars except a few toys and cheap opera glasses employ prisms as part of their optical system. There are two reasons for this. The first is to correct the image orientation, which as it comes through the objective lens is turned upside down and reversed. The second is to allow the binocular to be shorter than it otherwise would be to gain sufficient magnification.
You can make a simple telescope—as Galileo did—with nothing but a convex objective lens and a concave eyepiece that can be moved back and forth to focus. The image will be erect and the right way round with this simple arrangement, and those toy binoculars and opera glasses are made this way. But the Galilean telescope and binoculars are extremely limited in magnification, and the image blurs quickly toward the edges.
The Keplerian telescope (for Johannes Kepler) was an improvement in that it used two convex lenses, allowing longer focal lengths and much higher magnification. However, the image in the eyepiece or ocular was reversed and upside down—not an issue for astronomy, but definitely so for terrestrial viewing. Adding an additional convex lens rights the image, and the classic collapsible “spyglass” of pirate movies and highland stag hunts is made this way. They are, however, necessarily long for their magnification. A binocular made this way would be unwieldy to say the least—thus the prism.
Prisms can be used to do different things. We’ve all seen dispersive prisms—the triangular versions that break up white light into its lovely constituent colors. Binoculars employ reflective prisms, which at their most basic work on the principle of internal reflection—light entering perpendicular to one surface is reflected and redirected off the angled surface behind it. As long as the light hits the back surface at what’s called a critical angle, no mirrored surface is needed; perfect reflection is achieved at the glass/air interface. You can see this effect by looking up at the surface of an aquarium at an angle that turns it into a mirror.
The first prisms used in binoculars (by a smidgen) were porro prisms, named after their inventor, Ignazio Porro; they’re still employed today. In binoculars, two porro units are cemented together at right angles to each other in each optical tube, which gives binoculars in which they are used the classic offset configuration, where the objective lenses are set outboard of the oculars (or, in some compact binoculars, inboard). The advantage to porro prisms is that they obtain perfect internal reflection using nothing but glass—no mirrored surfaces are needed. This gives them intrinsically better light transmission and clarity than Schmidt-Pechan roof prisms (see below). Additionally, porro-prism binoculars with objective lenses set outboard of the oculars can render a slightly better three-dimensional view. Disadvantages include poor close focusing ability ( also an artifact of the wide-set objectives) and more difficult weather sealing, along with greater bulk and, frequently, weight.
A porro prism unit as used in a bincocular
Roof prisms overcome those bulk and weight issues. The most commonly used roof prism configuration in binoculars, the Schmidt-Pechan, comprises two prisms separated by a minuscule air gap. In part because incoming light rays are “bounced” more times in a tighter space than in a porro-prism instrument, roof-prism binoculars are more compact. Note in the diagram how the incoming and outgoing rays are in line; thus roof-prism binoculars can be configured as straight tubes, saving the weight as well as the bulk of the offset tubes.
But there are downsides. Collimation—that is, the internal alignment—of roof prisms is critical and expensive to replicate consistently and durably. Also, every time light is reflected it loses a tiny bit of brightness, and one of the prism surfaces in the Schmidt-Pechan design reflects at less than the critical angle, so it must have a reflective coating applied. Finally, the internal reflections of the Schmidt-Pecan design first separate the light beams, then recombine them, which causes slight polarization of the beams, reducing contrast. Manufacturers counter these issues with phase-correction coatings, which porro prisms do not need, and by using either a silver or, much better, dielectric coating on the reflective surface. Schmidt-Pechan prisms employing phase-correction coatings and a dielectric reflective surface come very close to the performance of a porro prism. Nevertheless, making a high-quality roof-prism binocular is more complicated and more expensive than making a high-quality porro-prism equivalent.
Another type of roof prism, the Abbe-Koenig, does boast perfect internal reflection and has no need for reflective coatings (although it still requires phase correction). However, as you can see in the diagram here, Abbe-Koenig prisms are significantly longer than Schmidt-Pecan prisms, and are rarely used except on large binoculars such as an 8x56, where light weight and compact size are less important than ultimate light-gathering power.
The two binoculars on the left employ Schmidt-Pechan roof prisms; the two on the right, Abbe-Koenig roof prisms.
So far this sounds like an open-and-shut case for porro-prism binoculars. However, if you’ve ever carried full-size binoculars around your neck for an hour or two, or looked through them for more than a few second at a time, you’ll be well aware of how much difference a few ounces can make in preventing fatigue and image shake. A roof-prism binocular of the same objective and magnification can weigh several ounces less than an equivalent porro-prism instrument, in addition to its reduced bulk. Roof-prism binoculars are generally easy to use one-handed when necessary—often impossible with porro-prism instruments. For this reason (in addition to their easier weather-sealing and close-focus capabilities), virtually all ultra high-end field binoculars are roof-prism models. And manufacturers have developed roof prism technology to the point that the differences between high-quality examples of each type would be difficult, if not impossible, for a casual viewer to detect. In fact, given the relative lack of development in porro-prism technology in the last couple of decades, I’d wager that the best current roof-prism binoculars are equal or superior in terms of light transmission and clarity to the best available porro-prism binoculars.
With that said, do keep in mind that manufacturing a bright and durable pair of roof-prism binoculars is significantly more difficult than doing so with porro prisms. If you are on an extremely limited budget, you might find you can get noticeably better quality out of the latter than the former, for the same price.
Not all prism glass is equal. The very best we have today is called BaK4, for BaritleichKron or Barium Crown, a glass made by the German company Schott AG. It has a very high refractive index (1.569 if you want to know, which is, like, really high, to be technical). Many cheap binoculars claim to be made from this glass when in fact they are not; there is essentially zero enforcement of such marketing lies. The manufacturer’s reputation—and price—is nearly the only way to be sure you’re getting Schott BaK4. And even given that, manufacturing tolerances, coatings, and a dozen other parameters will make or fail to make a high-performing prism.
The next critical aspect of binocular design is the lens coatings. Look at the objective lenses of your binoculars and you’ll notice a definite tint—purplish, greenish, reddish, more or less of each depending on the brand. Why is this?
All glass reflects some light—look at a piece of window glass and you’ll see this. Furthermore there is a reflection both when the light enters the sheet of glass—or a lens—and when it exits the back of it. This reflection can reduce the amount of light making it all the way through a lens by ten percent or more. Multiply that by the several lenses in a binocular and the light fall-off is severe. In 1886 the 3rd Baron Rayleigh, John William Strut (who would win the Nobel prize in physics in 1904), found to his surprise that old, slightly tarnished glass he tested transmitted more light than new, clear glass. This led to the development of specialized anti-reflection coatings, introduced by the Carl Zeiss company just prior to WWII. In one leap of technology, the total light transmission through a typical 8x30 binocular increased from 50-60 percent to 70-80 percent. Today’s high-end binoculars are fully multi-coated—that is, every glass surface has an anti-reflection coating comprising up to seven layers—and total light transmission is well over 90 percent. However, like BaK4 prisms, “fully multi-coated” can mean a lot or not very much at all. Simply put, a $150 Chinese binocular with “fully multi-coated optics and BaK4 prisms” will not have the same stuff inside as a $2,000 binocular with “fully multi-coated optics and BaK4 prisms.”
Next in line is the clarity and contrast of the image controlled by the objective lens or lenses, the focusing lens behind it, any field-flattening lenses behind the prisms, and the lenses comprising the ocular. Careful grinding/polishing of high-quality glass will ensure a crisp image, but color rendition raises its own issues.
Remember the dispersive prism and its colorful rainbow of colors? To some extent this happens with light passing through any lens. The different colors of the spectrum bend at slightly different angles, and if they are not brought together somehow by the time they reach the eyepiece, the image will display a fringe of spurious color around it—almost invariably blue, because blue light is more sharply diffracted then yellow or red.
Chromatic aberration, as this is known, can be reduced using an achromatic objective lens, comprising two layers of different types of glass that together reduce diffraction, and/or with aspheric lenses, which accomplish the same thing by altering the shape of the lens, and/or with extra-low-dispersion (ED) glass, which doesn’t diffract as much to begin with. As with everything we’ve discussed, this adds to the cost of the binocular as well as the quality of the image.
The best binoculars in the world can be ruined if any moisture is allowed inside. Fogging obscures the image and damages coatings, and fungus or mold will completely destroy the instrument, necessitating a complete disassembly and restoration at best. For this reason good binoculars are fitted with O-rings to seal out moisture, and then purged of air with pure nitrogen (sometimes argon) at slightly higher than atmospheric pressure. These gasses are dry, cannot fog, and do not support mold or fungus. Better binoculars incorporate better seals yet retain ease of focusing (the focusing mechanism is one of the most vulnerable entry points for moisture).
Many high-end binoculars now incorporate a field-flattening lens behind the prism, designed to increase edge-to-edge sharpness. This is especially useful for those who use binoculars on a tripod for long periods, scanning the entire field of view rather than moving the binocular around.
Is there anything else? Oh yeah, a lot of it. Proper internal baffling to prevent flare; shielding and blackening of the sides of the prisms to prevent spurious light entering them; reinforced housing of the prisms to prevent de-collimation; proper size of the prisms to capture all available light; grooving the hypotenuse face of the prism to control abaxial rays. Construction of the housings—aluminum, or lighter and more expensive magnesium? Hydrophobic objective-lens coating? You get the picture, and perhaps begin to see why the price of two binoculars that are to outward appearances identical might have price tags that differ by an order of magnitude.
The best binoculars available today offer brilliant, sharp, true-color views comfortable for hours of viewing without eye strain; complete weather sealing; shock-proof construction; light weight, and a lifetime guarantee—often transferable. A premium binocular represents a considerable investment that will repay itself every time you use it, and continue to do so for decades.
Next up: Choosing the best for your needs.
Companion workshop: View our Field Arts workshop on optics, with Swarovski’s Ben Lizdas joining us for a Q&A, here or view below.
Antigravity’s new LiFePO4 batteries
One morning in the early 1990s, when I was still leading sea kayaking tours in Mexico, I found myself stranded on a remote beach at the end of a tour with a suddenly dead Sears Die-Hard battery in my FJ40 (this was before I’d ever heard of a dual-battery system). Rather embarrassed, I got a jump start from a client, sent everyone home ahead of me, then drove the entire way back to Tucson, towing a trailer full of kayaks, without turning off the engine. (It took some doing to convince the U.S. customs people I wasn’t just preparing to do a runner after I was pulled into the inspection lane. In an FJ40 pulling a trailer? They could have caught me on foot.)
Not the best place for battery failure.
The battery was well within its pro-rated warranty period, so once home I got a new one for around half price. But that really wasn’t the point, was it? If I’d sent everyone home before discovering the dead battery, I would have had a very long walk to the nearest fishing village—I certainly couldn’t have push-started an FJ40 solo in beach sand. I was also aware of other circumstances I’d been in that could have been as bad or worse if a battery had failed. My experience with Die-Hards—then the much-hyped premier battery on the market—was that they lasted an average of two years before failing, usually abruptly.
There had to be a better way. I did some research (remember when that took actual effort?), which led me to news of a recently available type of über SLA (sealed lead acid) battery called a “gel cell” or AGM (for absorbed glass matt). A company called Optima made them, and the one that suited my FJ40 was around $130 special order—an outrageous price when a Die-Hard went for $55. I winced and ordered one anyway, and that first red-top Optima lasted six years before—very politely—showing signs of weakening. Thus, not only did it prove less expensive in the long run, it saved me at least two battery failures that could have happened anywhere. The AGM battery (I switched to Odyssey later on) became my standard battery configuration—as did a dual-battery system.
Twenty five years later there is a new über battery on the market, with a price as startlingly higher than a typical AGM as that Optima was higher than the Die-Hard. Does the lithium iron phosphate, or LiFePO4, battery represent as much of a leap as the AGM did? Can an overlander on a modest budget justify spending $800 or more for a single battery?
I’d like to find the answer—for myself as well as readers who might be contemplating the upgrade. So I’ll be installing a new dual-battery system in our Land Cruiser Troop Carrier, using one of Antigravity’s new DC-100-VI deep-cycle LiFePO4 batteries, along with one of their already introduced LiFePO4 starting batteries.
Not your father’s car battery . . .
I’ve been a fan of Antigravity’s products since their pioneering, pocket-sized Micro-Start jump-starting unit, which I embraced very early on and with which I’ve astonished more stranded motorists than I can count, including dozens at several Overland Expos who immediately headed for the Antigravity booth to buy their own. I also astonished, and rather horrified, Scott Schafer, the founder of the company, when I called to tell him that Tim Scully and I had successfully daisy-chained three Micro-Starts and performed an excellent field weld. That function was definitely not within his original design goals—yet we still have all three of those units and they still work perfectly.
NOT recommended!
Given that history, I have every expectation that Antigravity’s LiFePO4 automotive batteries will display similar quality. But I had to promise Scott I wouldn’t try welding with them.
So what’s all the fuss about LiFePO4 technology?
Most articles covering lithium batteries (to use the convenient shorthand) immediately mention the weight savings. And that aspect is impressive—The 100-amp-hour deep-cycle battery Antigravity sent me weighs 30.5 pounds; an equivalent Odyssey AGM unit weighs 69.5. Switching to a dual-battery system employing both starting and deep-cycle lithium units will, in our case for example, completely offset the weight of our 60-liter National Luna fridge-freezer. With a case of beer inside.
Thus there is an obvious advantage in energy density to the lithium battery. However, it’s even greater than the weight difference implies.
Consider a deep-cycle AGM battery and a deep-cycle LiFePO4 battery, each rated at 100 amp hours. While that seems equivalent on the surface, in delivery it is not. As soon as a draw is placed on an AGM battery, its voltage begins to drop in a more or less linear fashion. Once that voltage drops below 10.5 or so, a 12V appliance such as a fridge will shut itself off. At that point the battery retains nearly 50 percent of its theoretical energy, but it is unavailable to a 12V appliance because the voltage is too low.
By contrast, the energy delivery of a LiFePO4 battery is a nearly flat line that remains above 12 volts until the unit is almost fully discharged, at which point it drops off rather rapidly. Thus you get far more actual available power from a lithium battery than you would from an “equivalent” AGM.
But that’s not all. A LiFePO4 battery is also capable of accepting a charge at three to four times the rate of an AGM battery. A typical AGM in a vehicle can take as long as 10 hours to recover from a completely discharged state. A LiFePO4 battery can be recharged completely in four. And while an AGM battery typically can withstand around 300 such discharges, a lithium battery can withstand around 2,000—which brings up the last comparison: lifespan and amortized cost.
Currently a high-quality LiFePO4 battery costs two and a half to three times as much as a high-quality AGM battery from a manufacturer such as Odyssey. However, it appears likely the lithium battery will outlast the AGM battery by about the same factor—if not more. Thus over time the LiFePO4 battery should recoup its cost—and that doesn’t count the hassle of having two or three AGM batteries fail in that time. On an amp-hour basis, there’s no comparison—the LiFePO4 delivers far more energy over its lifespan.
(This obviously assumes that you keep your vehicle for a long time, or that you swap the lithium batteries from old truck to new truck, and say, “Get your own!” to the buyer of the old one.)
There are other advantages. For example, the Antigravity lithium starting battery has a built-in wireless jump-start feature. Leave the lights on or in some other way run down the battery, and it will shut itself down while retaining sufficient starting power to start the vehicle. Just tap a button on a wireless key fob to activate it; you don’t even need to open the hood. (You can also hit the button on the battery itself.)
So . . . end of argument? Not quite.
The bête noire of the LiFePO4 battery is temperature, or rather temperature extremes. Most lithium automotive batteries, including the Antigravity unit, can be used (i.e. discharged) at temperatures down to -20ºC (-4ºF). However, they cannot be charged at temperatures lower than about 0ºC (32ºF). The Cliff’s Notes reason for this is that, when recharging at temperatures above 0ºC, the lithium ions are absorbed by the porous graphite that comprises the anode—the negative side of the battery. Below this, the lithium instead begins sticking to the outside of the graphite, a process called lithium plating. This not only reduces efficiency but can permanently damage the battery.
Keep in mind that these critical numbers represent the internal temperature of the battery, not the ambient air temperature. As the outside temperature drops, the internal battery temperature will lag significantly. A night that drops to freezing or even several degrees below is unlikely to chill the internals to critical level—and we never charge batteries at night anyway. Also, drawing power from the battery—even for efficient LED camper lights—raises its internal temperature somewhat.
Fortunately, each Antigravity battery incorporates a sophisticated battery management system (BMS), which prevents charging when the temperature drops too low. So you don’t have to worry about damaging the battery; however, this aspect might be important if you camp for long periods in persistently below-freezing weather. Antigravity is also preparing to introduce a LiFePO4 battery with an automatic internal heat source that runs off the battery itself, as some other manufacturers have done. We don’t feel the need for this in our application—for Roseann, the words “snow” and “camping” never appear in the same sentence.
(As usual, clever owners who adopted LiFePO4 technology ahead of the rest of us have figured out their own hacks to deal with the low-temperature issue. Some have combined a simple temperature-controlled switch such as this with an inexpensive 12V heating film like this.)
(Another parenthetical aside: While researching all this I came across an article detailing the efforts of NASA scientists to extend the low-temperature parameters of lithium batteries—of obvious importance when sending them into space. The article noted that engineers had significantly extended these parameters by using an electrolyte formation of—ready?—1.O MLiPF6EC+DEC+DMC+EMC (1:1:1:2 v/v) and 1.O M LiPF6 EC+DEC+DMC+EMC (1:1:1:3 v/v). If they’d only called me I would have suggested that in the first place.)
Very high temperatures can also affect LiFePO4 performance. The listed upper limit for discharging the Antigravity deep-cycle battery is 55ºC (131ºF). For this reason, some owners install their lithium “house” batteries inside the vehicle or camper rather than in the engine compartment. This has the doubled effect of keeping the battery warmer on cold nights, especially if any kind of heat source is used in the camper for the comfort of occupants.
I’ve decided to take a slightly bolder approach, partially as an experiment to see the effects of different installations. Our Troopy has the stock dual-battery tray in the engine compartment, and I plan to mount both batteries there and monitor the temperature extremes they experience, using (in part) Racetech stick-on temperature strips, which record the high temperatures reached on the surface to which they’re attached.
The other part? That will be courtesy the built-in Bluetooth connectivity of each battery, which will enable me to monitor, through my iPhone:
Battery voltage
Current/amps going in or out
Watt output
State of charge percentage (SOC)
Interior temperature
Individual cell voltage
Number of cycles the battery has experienced
In addition to that internal temperature readout, the state of charge in percent will be especially helpful—given the LiFePO4’s characteristic of maintaining flat voltage delivery, it’s difficult if not impossible to ascertain the state of charge by voltage alone, as on an AGM battery.
Our Troopy’s battery tray is in the back right corner of the engine compartment, looking from the front of the vehicle. The 1HZ diesel’s exhaust manifold is on the opposite side of the engine, which will help mitigate heat gain. I also plan to have fabricated a metal heat shield that will block the inside faces of the batteries, to help even more. I’ll add some sort of heat barrier to the sheet metal as well. In a few months the southern Arizona summer should give us some solid data on the effects on both batteries; then we can re-evaluate their placement.
I’m going to take the opportunity to replace the existing cables and terminals with new ones, and clean up the rather tangled routing resulting from adding on the winch, driving lamps, and National Luna isolator.
There is one other cost factor to consider when considering a LiFePO4 battery. As I mentioned, a lithium battery has a very different charging profile from an AGM battery. Thus, for the deep-cycle unit I’m installing in the Troop Carrier, I’ll need to swap the existing DC-DC charger, designed for AGM batteries, for one that includes a setting for lithium batteries and is suitable for routing and managing both alternator and solar power to the Antigravity battery. Such units can run from about $300 to $400. This is pretty much a one-time outlay, but it still needs to be factored into the initial investment.
I’ll be doing regular updates here as soon as the starting battery arrives.
ARB Solis driving lamps
I’ve written before about my admiration for ARB’s Intensity driving lamps. We have a set on both our Troopy and the FJ40, which have saved us from, respectively, kangaroo and cow strikes on remote roads on two continents.
The lamps on the Troopy are first-generation Intensity lamps, and I would have considered it difficult to improve on them, but in 2019 ARB introduced a V2 version with 35 percent more power. I put those on the FJ40, and indeed they are noticeably (even) brighter.
But now, just a year later, ARB has introduced an entirely new lamp, the Solis.
At first glance the Solis looks like another update of the existing, larger Intensity V2 AR32 (the Solis at present only comes in the larger size; there’s as yet no equivalent to the smaller V2 AR21). But there are actually quite a few design modifications, one major difference—and one huge difference.
The 32 in the V2 Intensity name refers to the number of Osram LED emitters in the lamp. The Solis ups this to 36, and, moreover, splits their duties. There are 30 4-watt emitters in the perimeter of the lamp, and 6 10-watt emitters in the center, to give some extra reach in the center of the beam, even in the flood-patterned model. As a result, the performance of both the spot and flood model of the Solis exceeds the V2 AR32 Intensity by about 20 percent.
The major difference is that the Solis is dimmable over a five-stop range, via a remote-control wand. On the surface it might seem pointless to build a 165-watt Osram-powered driving lamp and then make it dimmable, but in fact there are many situations in which you want more light than your high beams can provide, but less than the Solis’s full 9,500 effective lumens. On winding roads lined by trees, for example, the glare from full-powered lamps can be a hindrance. The reflection from road signs can also be nearly blinding at times. If there’s a lot of dust or moisture in the air you might be better off with less reach.
Remote controls the five-step dimming function
And the huge difference? That’s the price. The V2 AR32 Intensity lamps retail for $783 each. The Solis lamp of the same size retails for $558. Per pair. With a wiring loom.
What explains this? You might have already guessed. The Intensity V2 lamps are manufactured in the United States by Rigid Industries. The Solis was engineered in Melbourne, Australia, and is manufactured in China.
I’m a bit conflicted about this, but not much. Sure, I’d prefer the U.S.-manufactured items and I’m glad those are the ones we have. But I’m fully aware that not everyone can spare that kind of change for driving lamps, and those people are prime targets for the many knock-off lamps that replicate the look of the Intensity lamps without their build quality. The combination of Chinese manufacturing . . . let’s call it “efficiency” . . . with, one fully expects, ARB quality control, should provide a reliable alternative for those who fainted when they read that first price above.
Are there any other cost-cutting approaches? Not that I can see from photos or specifications. The Solis has a cast-aluminum body, a one-piece reflector, a polycarbonate lens, and is submersible to three meters. It echoes a bit of the Intensity look with two red trim sections, but these can be swapped out for black if you prefer a more low-profile appearance.
My hope is that both these lines survive. I’m happy they’re both there, but I’ll be disappointed if sales of the U.S.-made product simply disappear in the face of the in-house Chinese competition.
I’m hoping to get a pair of Solis lamps to actually test soon. In the meantime, ARB is here.
Hint: When using “Search,” if nothing comes up, reload the page, this usually works. Also, our “Comment” button is on strike thanks to Squarespace, which is proving to be difficult to use! Please email me with comments!
Overland Tech & Travel brings you in-depth overland equipment tests, reviews, news, travel tips, & stories from the best overlanding experts on the planet. Follow or subscribe (below) to keep up to date.
Have a question for Jonathan? Send him an email [click here].
SUBSCRIBE
CLICK HERE to subscribe to Jonathan’s email list; we send once or twice a month, usually Sunday morning for your weekend reading pleasure.
Overland Tech and Travel is curated by Jonathan Hanson, co-founder and former co-owner of the Overland Expo. Jonathan segued from a misspent youth almost directly into a misspent adulthood, cleverly sidestepping any chance of a normal career track or a secure retirement by becoming a freelance writer, working for Outside, National Geographic Adventure, and nearly two dozen other publications. He co-founded Overland Journal in 2007 and was its executive editor until 2011, when he left and sold his shares in the company. His travels encompass explorations on land and sea on six continents, by foot, bicycle, sea kayak, motorcycle, and four-wheel-drive vehicle. He has published a dozen books, several with his wife, Roseann Hanson, gaining several obscure non-cash awards along the way, and is the co-author of the fourth edition of Tom Sheppard's overlanding bible, the Vehicle-dependent Expedition Guide.